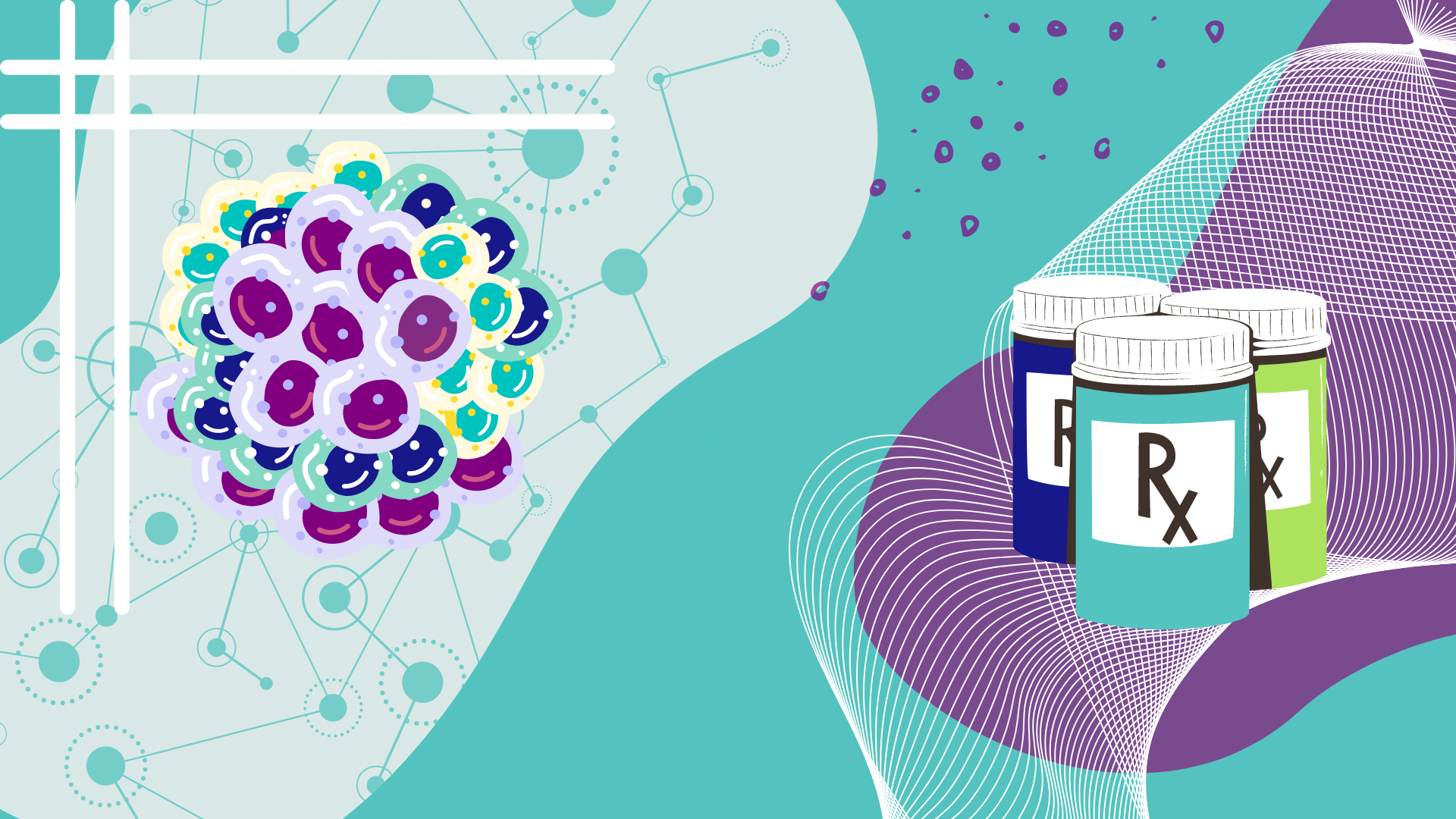
Miniaturised Replicas of Tumours are Changing How we Look at Cancer
Juli Udayani is a research assistant at the Faculty of Medicine, University of Helsinki. She works on brain cancer progression and metastasis research.
This article is part of the intersections theme.
edited by Kenia and carmine d’amico, edited & reviewed by marie-noëlle paludetto, and illustrated by valeria ariotta.
As science and technology continue to advance, researchers are discovering innovative ways to tackle complex problems, including the fight against cancer. One of the breakthroughs is the intersection of biomaterial science and cancer biology, which has led to the development of cancer organoids. What are these 3D cancer miniatures that mimic the architecture and pathophysiology of a patient’s tumour and offer new avenues for studying cancer and testing anticancer drugs? Welcome to our mini-series of “Cancer Encounters”.
What is cancer?
“Is there already a cure for cancer?”, my relative asked me. I hesitated. As a researcher studying brain cancer, I know that the answer to that question is not as simple as a yes or no. According to the Global Cancer Statistics (GLOBOCAN) study 2020, 19.3 million new cancer cases and almost ten million cancer deaths occurred in 2020. Looking ahead, projections indicate that the number of new cancer cases worldwide may increase to 28.9 million by 2040 with a 15.9% rise in deaths among individuals under 50 years old. These staggering numbers highlight the complex nature of cancer, which manifests in various tissues and has diverse causes, mechanisms, and outcomes. And here lies one of the biggest misconceptions around cancer: it is, in fact, not a single disease.
Cancer is a diverse group of diseases that share a common feature: uncontrolled growth of cells due to numerous abnormal changes in their genes. Such changes, called genetic anomalies, can arise from a variety of factors, including familial predisposition, errors during cell division, and exposure to harmful substances that might damage DNA.
Since the early 2000s, scientists have been summarising the attributes of cancer known as “Hallmarks of Cancer”, a collection of ten essential characteristics that define how cancer cells behave and interact with their environment. They provide a framework for understanding the complex biology of cancer and guide researchers in developing new approaches for cancer diagnosis, treatment, and prevention. So where do we stand now?
Traditional cancer models
Since the 1990s, the number of new anticancer drugs has doubled and novel treatments targeting the tumour and its environment have emerged. There are many reasons for this, including the increase in time and resources allocated to cancer research as well as improved regulation on clinical trials, but one crucial contributor has been the availability of reliable models.
Models are simplified representations of real-world systems or phenomena used to understand and predict their behaviour. Injecting patient-derived cancer cells into lab animals, known as animal models, allows researchers to test the efficacy and safety of selected drug candidates. However, animal models are expensive and require laborious handling. They also have a different biology that may not truly represent the tumour and its environment in human patients. As a result, only around ten percent of novel drugs that passed animal preclinical studies successfully enter the market. These limitations, along with ethical considerations, emphasize the need for alternative, cost-effective models that accurately represent patients’ original tumours.
Organoids, miniaturised replicas of tumours
In the exciting field of biomaterial engineering, scientists have made remarkable progress in developing a new type of model called cancer organoid. Organoids serve as miniaturised replicas of actual tumours, much like the intricate models architects create when designing buildings. Why is this important? Because organoids allow scientists to construct a “prototype” tumour composed of various cell types, including non-cancer ones, that mimics the original architecture of patients tumours. This is possible because of the support of an extracellular matrix, commonly referred to as ECM (keep this acronym in mind because, as you will soon read, it’s more important than you think!). But first, let’s watch a short video.
As you saw in the video, organoids are grown in the laboratory and allowed to self-organise, enabling researchers to study cancer growth and migration within the body and evaluate anticancer therapies. Compared to animal models, cancer organoids better represent the native cancer characteristics in humans because they are fully formed by human cells of the original tumour. They are easier, cheaper and faster to use than animal models, making them useful for the early screening of numerous drugs and to develop new and better therapies to fight cancer. Organoids are also a great way towards limiting animal testing: as alternative models, they allow to reduce the number of animal experiments according to the 3Rs (Replace, Reduce, Refine) ethical principle in the animal research framework.
But back to ECM, a complex network of proteins and other molecules that surrounds all cells in our body and holds tissues together. Understanding the relationship between the composition of the tumour ECM and its physicochemical characteristics is crucial because ECM doesn’t only serve as the structure for the cancer cells to grow but also influences their mobility and interaction with other cells.
We have recently discovered that the ECM in tumours is stiffer compared to the ECM in healthy tissues due to extra collagen. The increased stiffness of the tumour tissues helps the cancer cells to stick together and spread to other parts of the body through the blood and lymphatic systems. In addition, certain components in the ECM, like laminins, interact on the surface of cancer cells with receptors that can activate pathways promoting cell growth and adhesion.
These findings highlight the importance of studying the tumour ECM to develop reliable organoid models and decipher mechanisms of tumour progression, providing more insights for targeted therapeutic approaches. For years, cancer researchers have been using ECM isolated from mice tumour tissues to create organoids. However, there is significant batch-to-batch variability in the characteristics of the animal-derived ECM, leading to organoids with variable features. To overcome this problem, scientists are starting to develop their own ECMs in their labs, defining and optimizing their characteristics by combining various chemicals. For example, they can fine-tune the material stiffness by adding certain compounds like polyethylene glycols (PEG). This approach enables scientists to produce ECM that accurately mimics the properties of different types of cancer, such as intestinal or brain cancer.
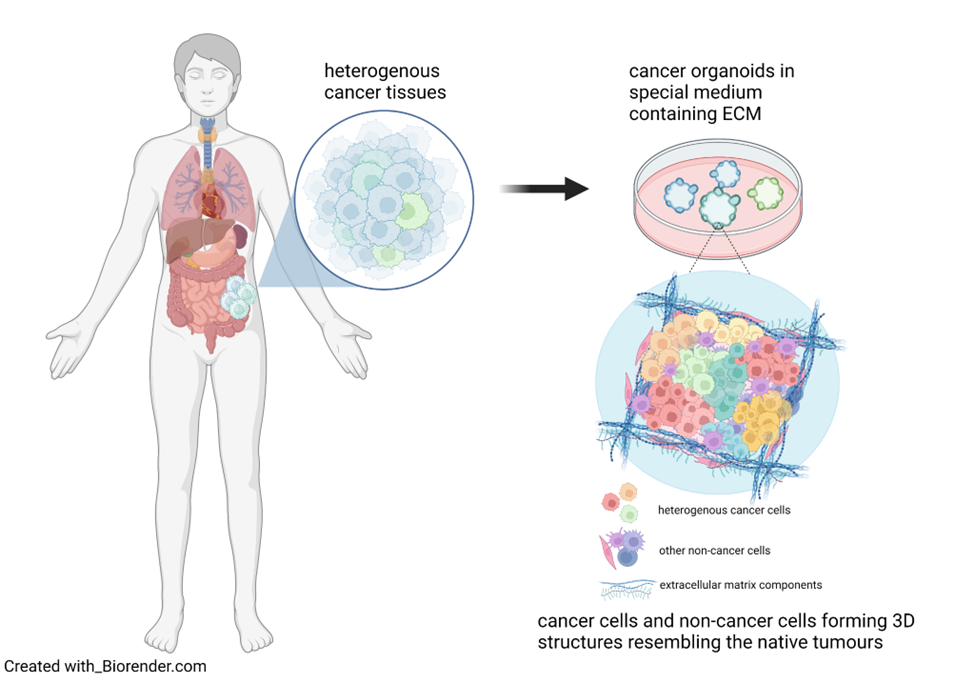
The future of organoids
What do organoids hold for the future of cancer research? Overall, organoids hold great promise for advancing our understanding of cancer and developing new treatments, mainly because they preserve the native complex features of patients’ tumours and are more economical at a large scale. Using these organoids as cancer models, researchers can accurately learn how cancer cells grow and spread in the body and identify precise targets to kill cancer cells effectively. Moreover, organoids are useful in drug development, allowing scientists to test numerous drug candidates quickly and cost-effectively.
As promising as they sound, organoids still face several challenges including their limited long-term survival in the laboratory and technical variability arising from the lack of standardised production techniques. To solve these obstacles scientists will have to keep improving their knowledge and technologies to prolong organoids’ lifetime and to standardise the culture techniques, including the source tissue, processing protocols, and medium composition.
Developing anticancer drugs is a long road, typically taking at least ten years, but continued collaboration between biomaterial science and cancer biology to improve organoid models holds great potential in accelerating precision cancer medicine. There will probably never be a “one cure for all” solution, but through the intersection of different research fields, we might be able to create new tools and ensure that we can find as many solutions as necessary.
_____________
In the next article about cancer encounters, we will discuss how computer science helps researchers predict cancer cells’ behaviour and interaction with other cells. Do researchers still have to perform experiments in the lab to study cancer, or will computerisation be able to replace cancer experiments?
references
FIN3R. (2023, June 5). 3R centre Finland. FIN3R. https://fin3r.fi/en.
Gentilin E, D’Angelo E, Agostini M, Astolfi L. Decellularized normal and cancer
tissues as tools for cancer research. Cancer Gene Ther. 29(7), 879-888 (2022).
Gjorevski N, Lutolf MP. Synthesis and characterization of well-defined hydrogel
matrices and their application to intestinal stem cell and organoid culture. Nat
Protoc. 12(11), 2263-2274 (2017).
Han LH, Tong X, Yang F. Photo-crosslinkable PEG-based microribbons for forming 3D
macroporous scaffolds with decoupled niche properties. Adv Mater. 26(11), 1757-62
(2014)
Hanahan, D. Hallmarks of Cancer: New Dimensions. Cancer Discovery 12, 31–46
(2022).
Kratochvil, M. J. et al. Engineered materials for organoid systems. Nat Rev Mater 4,
606–622 (2019).
Kim J, Koo BK, Knoblich JA. Human organoids: model systems for human biology and
medicine. Nat Rev Mol Cell Biol 21(10):571-584, (2020).
Kinch, M. S. An analysis of FDA-approved drugs for oncology. Drug Discovery Today
9, 1831-1835 (2014).
LeSavage, B.L., Suhar, R.A., Broguiere, N. et al. Next-generation cancer
organoids. Nat. Mater. 21, 143–159 (2022).
Londoño-Berrio, M., Castro, C., Cañas, A., Ortiz, I. & Osorio, M. Advances in Tumor
Organoids for the Evaluation of Drugs: A Bibliographic Review. Pharmaceutics 14,
2709 (2022).
Lu, P., Weaver, V. M. & Werb, Z. The extracellular matrix: A dynamic niche in cancer
progression. Journal of Cell Biology 196, 395–406 (2012).
Mak IW, Evaniew N, Ghert M. Lost in translation: animal models and clinical trials in
cancer treatment. Am J Transl Res 6(2), 114-8 (2014).
Sung H, Ferlay J, Siegel RL, Laversanne M, Soerjomataram I, Jemal A, Bray F. Global
Cancer Statistics 2020: GLOBOCAN Estimates of Incidence and Mortality Worldwide
for 36 Cancers in 185 Countries. CA Cancer J Clin. 71(3), 209-249 (2021).
Xiao, W., Sohrabi, A. & Seidlits, S. K. Integrating the glioblastoma microenvironment
into engineered experimental models. Future Science OA 3, FSO189 (2017).