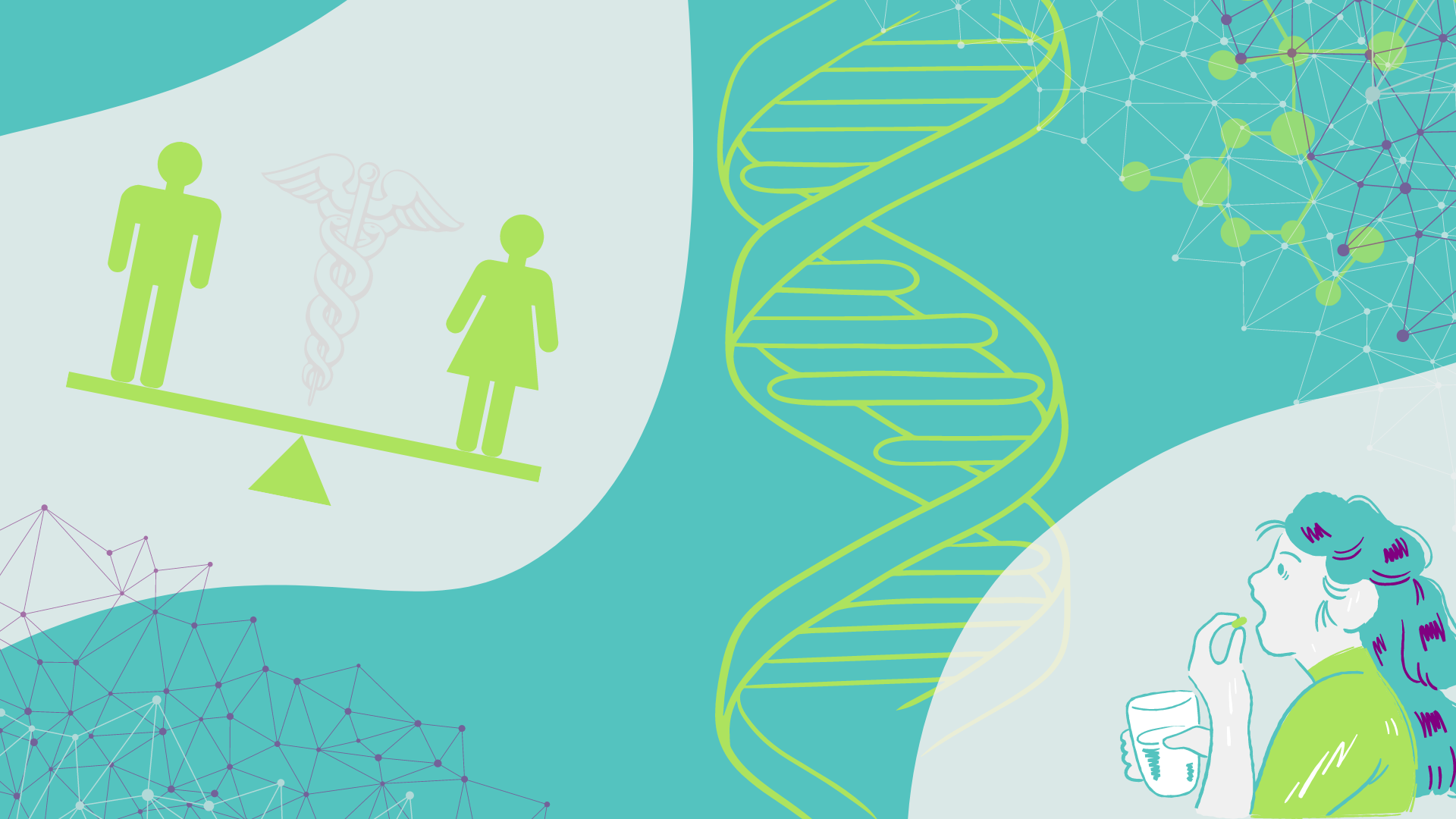
Ghosts in the universe
Dr. Tommi TenkaneN is a PhD, author, adjunct professor (docent) of theoretical physics
It was year 1970. In the picturesque town of Cambridge, UK, it was usual to see a wheelchair running across the winding streets – most of the time terribly fast. The person driving the wheelchair was a young man, who was later to become a renowned scientist and author whose name everyone knew. His name was Stephen Hawking.
By the beginning of the 1970s, Hawking had already made a name for himself among the scientific community by his publications on space-time singularities. In 1970, he was four years apart from deriving his most famous result, the evaporation of black holes, later to be known as Hawking radiation. However, that year he made another important discovery – with far-reaching consequences.
Hawking had studied black holes of various kinds and was interested in how they form. It was known that black holes can form when massive stars collapse at the end of their life cycle but the general theory of relativity did not seem to require that the massive progenitor is a star. All that was needed was sufficient density or compactness so that space-time could deform to form a hole which even light cannot escape. Where could there be such enormous densities, if not in the centres of collapsing stars?
In the early universe, concluded Hawking in 1970. He calculated that when the observable universe was compressed to a tiny volume during the Big Bang, large enough inhomogeneities in matter density could have led to formation of microscopical black holes. Because such black holes would not have stellar origin but could have formed in the Big Bang, they became known as “primordial black holes”.
Actually two Soviet scientists, Yakov Zel’dovich and his former student Igor Novikov, had presented similar ideas already few years before Hawking but they had made a crucial mistake in their calculations. They had neglected the expansion of the universe and obtained a result that in the end of their formation process primordial black holes should have grown enormously and be more than billion times more massive than the Sun. Because astronomers had not observed such black holes, their conclusion was that black holes could not have formed in the early universe.
The first publication Hawking wrote on primordial black holes was a rather speculative one. In 1974, however, he was able to robustly show with his PhD student Bernard Carr that if the conditions were sufficient, black holes could indeed have formed in the early universe and, more importantly, without having grown almost indefinitely. On the contrary, they showed that such black holes would often be very light in their class and could therefore differ from the usual, stellarmass or larger black holes. They would move slowly and be very difficult to observe. Could such black holes be interesting at all?
Black holes are not forever
“Perhaps the most important aspect of the conclusion that primordial black holes might have formed was that it motivated Stephen to consider the quantum effects of small black holes. Only primordial ones can be small enough for this to be important,” says Bernard Carr, now an emeritus professor in Queen Mary University of London, UK.
This notion led Hawking to his most famous result: when quantum field theory is applied to black holes, it predicts that black holes are in fact not perfectly black but radiate particles and light thermally. This curious phenomenon became known as Hawking radiation and it revealed that black holes are not forever but that they eventually evaporate into space. How fast this happens depends on the mass of the black hole: the more massive the object, the longer it takes.
The result implies that there is a lower bound on the mass of a black hole that can have survived until the present day if it formed in the early universe. The bound is around [latex] 10^{12} [/latex] kg, i.e. one billion tons, which equals the mass of a small asteroid – just compressed to a tiny volume. This means that maybe not all microscopic black holes have evaporated by today, given that they formed in the early universe in the first place. In that case, one question still remains: where are these black holes?
A ghost in the universe
Since the 1970s, it has been known that there is a ghost in the universe. On top of all the visible matter we can see, there must be invisible but massive matter everywhere in the universe. This ghost-like “dark matter” component must exceed the amount of ordinary, visible matter by a factor of five and it must have been there – everywhere – since the Big Bang. Moreover, it is known that this matter component cannot constitute of ordinary matter, such as atoms or known elementary particles, but it must be some new kind of matter.
This is known from a variety of astronomical observations ranging from galactic dynamics in our cosmic neighbourhood to precise measurements of characteristics of the cosmic microwave background radiation, which is also known as the afterglow of the Big Bang. This elusive matter component has puzzled astronomers and theoretical physicists for decades and had profound consequences also on the philosophy of science: what are the fundamental building blocks of nature and how have they come to be?
Theoretical physicists have come up with a multitude of possible explanations. Maybe dark matter is a new elementary particle? Or maybe it consists of some sort of a superfluid? Or maybe we just do not understand gravity well enough and, with a more fundamental theory of gravity at hand, no new matter components are needed?
At the moment we do not know the answer. Models where dark matter consists of new particles are by far the most studied ones, whereas modified gravity theories reside in the opposition. Yet particle colliders, such as the Large Hadron Collider in Geneva, Switzerland, and other carefully planned experiments have failed to discover a dark matter particle. The only thing that is clear at the moment is that there has to be something that explains the astronomical observations.
To better understand the nature of dark matter, one must ask what is needed for a matter component to explain the dark matter astronomers observe. First, it must be massive. Second, it must be “cold”, in cosmological jargon, meaning that it cannot move fast. Third, it must have had the correct abundance from the time of the Big Bang to the present day. Sounds something we have a candidate for, does it not?
Could black holes explain dark matter?
Primordial black holes are, in principle, perfect dark matter candidates. They are typically very massive compared to elementary particles, move slowly, and might have the correct abundance. Because they do not have stellar progenitors but – if they exist – formed at a very early stage in the history of the universe, they also are not ordinary matter turned dark. When it comes to explaining dark matter, that is a possibility that has been ruled out by observations.
The problem with primordial black holes, however, is that so far we have not detected any and the observational constraints for their abundance are becoming increasingly tight. Only around 10% of dark matter seems to be allowed to constitute of black holes, maybe even less than that.
Luckily, with the recent discovery of gravitational waves emitted when two black holes merge, astronomers now have a unique probe also on the physics of dark matter. In particular, a future observation of a black hole merger with one black hole lighter than the largest possible mass for a stable star, 1.4 Solar masses, would unambiguously imply it has a primordial origin, as otherwise it could not have formed. Such an observation would also prove that primordial black holes contribute to the observed dark matter abundance.
Other ways to test the primordial-black-hole-dark-matter hypothesis include detailed observations of gravitational lenses and the cosmic microwave background radiation. The increasingly tight constraints have made most physicists sceptical but the battle is still on-going. Astronomers and physicists alike are now revising the observational constraints and looking for new ways to test the scenario. Regardless of the outcome, it is simply wonderful that we are now entering an era when we can finally either verify or falsify the possibility that primordial black holes formed in the first place or, even more astonishingly, explain the dark matter enigma.
Sadly, Stephen Hawking is no more here to see that; he died in March last year. Hawking was widely celebrated and received multiple prestigious awards for his work on black holes but maybe over them he would have preferred to receive a proof of the existence of primordial black holes, for there is no better award for a theoretical physicist to see one’s predictions actually realised in nature. However, I guess we can take comfort in thinking that if primordial black holes were ever detected, that would be the last triumph to Stephen Hawking – this time from the cosmos itself.