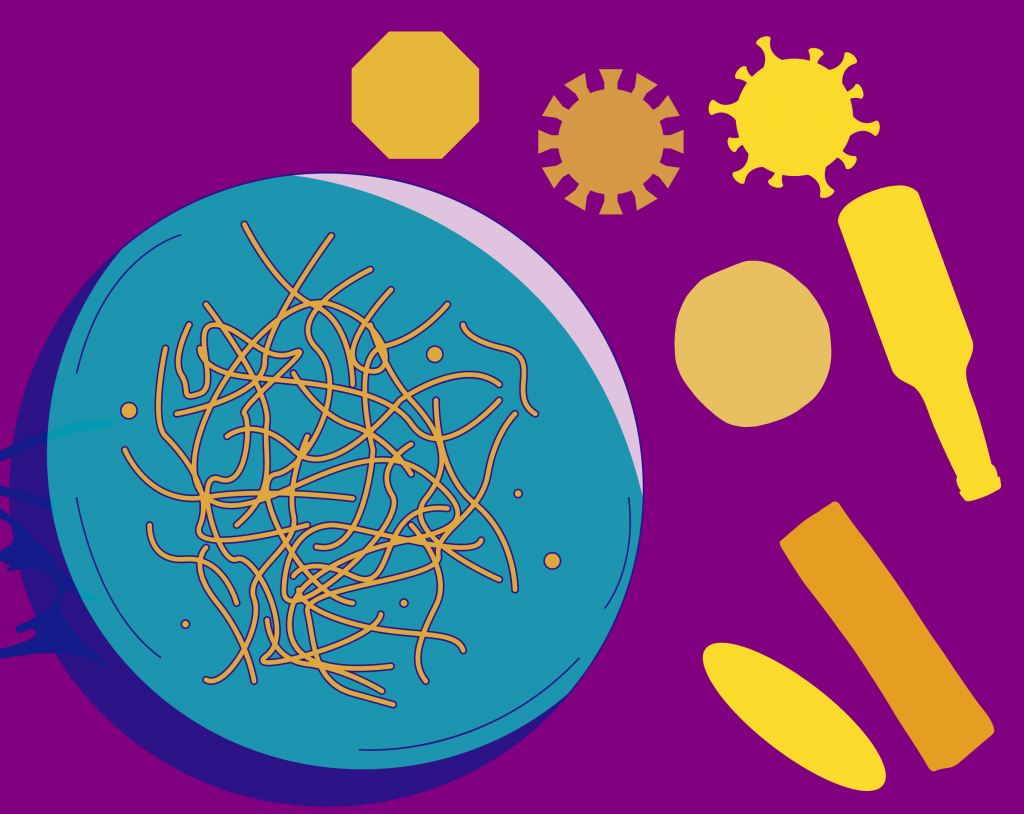
Nearly 3.5 billion years ago, our planet had extreme temperatures and was predominantly covered with water. The hostile environment was devoid of oxygen and high in methane. Around this time, an elegant and primitive life form slowly evolved to become among the first residents of planet earth. These single-celled microscopic beings have since thrived as our planet aged and newer and more complex life forms came into being. Though unknown by many, with the help of a few curious minds, a new chapter begins to unfold for this ancient life form somewhere in a lab in Paris.
It was around 9, a relatively cold and gloomy winter morning. The metro stations of Paris were already bustling as many rushed to catch the train in time for work. Miguel Gomez-Raya Vilanova, a 25-year-old Ph.D. researcher, having just arrived in Paris a couple of months ago, was eager to get into the train as it approached the platform. He had started working in the world-famous Institut Pasteur after being accepted into a highly competitive PPU (Pasteur-Paris University) postgraduate research program. Entering the institute’s 80’s style brick and stone building, Miguel was among the first to arrive in his lab. Grabbing his lab coat, he went on to check the overnight cultures of a peculiar organism that he had been growing for his research- the Archaea.
Though fundamentally very similar to bacteria, Archaea belong to a different branch on the tree of life. Life on this planet can be classified into three domains: one bacteria, other eukarya, which includes fungi, plants, and animals, and the third, Archaea. Like the avengers of the marvel universe, these archaea have their own repository of superpowers. Some groups of archaea, known as halophiles, can survive in highly saline conditions like the Dead Sea and the Great Salt Lake in Utah. Some groups of archaea called acidophiles live in hot, acidic waters, such as those found in sulfur springs. Some can thrive at pH levels similar to concentrated sulfuric acid. Some live in oxygen-deprived waters in the deep ocean. Some, known as thermophiles, thrive at temperatures as high as 110 °C and are found in hot springs, volcanoes, and deep-sea vents. On the other extreme, psychrophilic archaea survive even at –45°C. None of the other life forms on Earth, except a few notable bacterial species, can survive such extreme conditions. So how can Archaea?
Cells from all the organisms belonging to the archaea, bacteria, and eukarya domain consist of a cell membrane, similar to the outer walls of a fort, that encases all the cellular components. However, only plants, fungi, algae, and most bacteria and archaea, have yet another layer outside the cell membrane called the cell wall, an additional defense line. The chemical compositions of the archaeal cell wall and the cell membrane are different from those found in bacteria, plants and fungus. This provides archaea with some protection in the previously mentioned harsh environments. Further, archaea contain a variety of unique proteins that are resistant to extreme heat or acidity, further contributing to their survival in these extreme conditions.
Not all archaea prefer to live in otherwise uninhabitable territories. Many archaeal species live in our oral cavity, skin, and gut. Although currently no archaea are known to be pathogens, that is, to cause any human disease, studying them has proven crucial. The highly heat stable and unique proteins found in these organisms have huge application potential in industries, especially in biofuel production, mining, processing of coal and pharmaceuticals. Another more exciting application is in space research. There has even been a growing interest in studying whether these archaea can survive in outer space or mars-like conditions. These studies will provide clues to whether life is possible on other planets without the favorable life conditions of Earth. If archaea can survive in ‘dead zones’ on our planet where previously life was thought impossible, why not in space or on other celestial bodies in the cosmos?
Miguel’s journey studying tiny organisms began a few years ago in 2018, when he got the opportunity to work with bacteriophages, viruses that infect bacteria, during his Erasmus exchange studies at the University of Helsinki, Finland. During his few months researching bacteriophages, he got captivated by these tiny bacteria infecting viruses and decided to pursue his Masters in Microbiology at the University of Helsinki. His curiosity with these microscopic pathogens ultimately drew him to Paris after his masters.
Despite the gloomy weather on that particular day, Miguel was quite satisfied with his overnight cultures of the thermophilic and acidophilic archaeon Sulfolobus islandicus. A sense of optimism struck him as he measured the amount of archaea cells from the conical glass flasks using a machine called the spectrophotometer. He had prepared these archaeal cultures as a base to grow something much more peculiar. He was interested in a tinier pathogen that infected these single-celled archaea- the archaeal viruses.
Archaeal viruses are some of the most unique and unusual viruses known, including bottle-shaped, spindle-shaped, and two-tailed viruses. Even though less than 100 archaeal viruses are known, their distinctive characteristics have already gained some attention. For example, sulfolobus islandicus rod-shaped virus 2, also referred to as SIRV2, an archaeal virus that infects sulfolobus archaea, demonstrates an unusual behavior never witnessed before. Once infected with this virus, archaea form many seven-sided pyramid-like structures on their cell surfaces. At the end of the infection cycle, the seven sides of these pyramid structures open outward, like a blooming flower, generating a large opening through which assembled progeny viruses leave the cell.
Most known archaeal viruses have been isolated from hot water springs and highly saline lakes. Hence, these viruses have also developed many characteristics that help them withstand extreme heat, saline, or acidic conditions like their archaeal hosts. Viruses have shown promise as an ideal nanoparticle drug delivery platform for treating human diseases and as building blocks for different nanostructures. Compared to other human or animal viruses, archaeal viruses’ unique structure and extremophilic nature makes them suitable, for example, in effectively delivering a drug to our acid-rich stomach or in building nanomaterials that can withstand extreme heat. Despite the growing interest in archaeal viruses and their potential applications, currently, how these archaeal viruses interact with their hosts remains unexplored. It is crucial to obtain a thorough understanding of their biology before these virus-based nanoproducts can be implemented in practice. Miguel hoped that his research would contribute an additional piece to the unfinished jigsaw puzzle of our knowledge of the archaeal virus.
Back in the lab, as the day progressed, Miguel moved on to perform his next critical experiment. He infected the archaeal cells with SIRV 2 virus. Meanwhile, he thought about already preparing for further assays, which would help him assess the quality of the progeny virus production after a successful infection. He needed to establish a well-growing virus-host culture to effectively study how they interacted with each other. Inside the Petri dish, the virus started to recognize the archaeal cells and attach itself to the walls of the archaea. As the newly inoculated viral particles began their cycle of infection, Miguel had also taken one step further in his journey towards unraveling the mysteries of the archaeal virus-host interactions. In the future, results from his studies and many others will provide us with new insights into the hard-core archaeal lifestyle, the evolution of these rare viruses, and their potential applications.
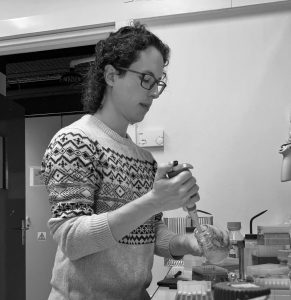
Acknowledgements
I would like to thank my dear friend Miguel for introducing me to the exciting world of archaea and archaeal viruses. Also for letting me interview him for this article and sharing with me the interesting aspects of his doctoral research.
Note
The name of the sulfolobus archea and the sulfolobus islandicus rod-shaped virus was recently changed to Saccharolobus and Saccharolobus islandicus rod-shaped virus 2 respectively.
REFERENCES
1) When did eukaryotic cells (cells with nuclei and other internal organelles) first evolve? What do we know about how they evolved from earlier life-forms? [WWW Document] (1999). URL https://www.scientificamerican.com/article/when-did-eukaryotic-cells/
2) Uldahl, K. B. (2015). Hyperthermophilic Archaeal Viruses as Novel Nanoplatforms (Doctoral dissertation, University of Copenhagen, Faculty of Science, Department of Biology, Functional Genomics).
3) Cabrera, M. Á., & Blamey, J. M. (2018). Biotechnological applications of archaeal enzymes from extreme environments. Biological research, 51.
4) Oren, A. (2014). Halophilic archaea on Earth and in space: growth and survival under extreme conditions. Philosophical Transactions of the Royal Society A: Mathematical, Physical and Engineering Sciences, 372(2030), 20140194.
5) Prangishvili, D., & Quax, T. E. (2011). Exceptional virion release mechanism: one more surprise from archaeal viruses. Current opinion in microbiology, 14(3), 315-320.